'Plastic pollution' is neither a new phenomenon nor a difficult concept to grasp. Today, even unborn babies could be exposed to micro-forms of plastics! I am not kidding! In 2020, the first evidence of microplastics in six human placentas was reported.
In 2022, researchers announced the presence of microplastics in human breast milk, causing widespread concern worldwide.
From the vast expanse of the 'Great Pacific Garbage Patch' (recognised in the late 20th century) to the microplastics in the remote and pristine Antarctic islands, from the plastics in the majestic beasts to the microplastics in human bloodstreams, plastics have spared no life and left no corner of the earth untouched since its invention in 1907.
Why Are Plastics So Popular and Widely Used?
What would you answer if asked, 'What makes plastic popular?' As a consumer, would you state the prevalent four? A cost-effective alternative (cheap!), versatile (adaptable), durable (long-lasting!) and water resistant.
I relate to it because I am a consumer as well. However, a conscious one!
Nevertheless, an engineer would highlight a plastic's wide-ranging properties, including but not limited to versatility, durability, insulation, lightweight, processing ease, and chemical resistance, making them suitable for various applications.
Can Plastics Be Replaced to Reduce Pollution?
Since its invention, plastics have substituted wood, glass, metal, rubber, paper, cardboard, ceramics, and leather in various industries worldwide. Given this extensive usage, can plastic be replaced to mitigate pollution and advance sustainability?
The answer lies somewhere between a no and yes.
Plastic pollution can be mitigated by strictly adhering to the widely known three Rs: Reduce, Reuse and Recycle. However, its success hinges on the willingness and responsibility of the consumers.
I refrain from presenting any statistics on plastic recycling for three reasons. One, most of the recycling data is outdated or country specific. Secondly, the assessment is flawed at many levels and does not provide a precise estimate. Third, it is not within the scope of this blog post.
Nonetheless, it is apparent that plastic recycling still has a long way to go. In many cases, cheaper production costs, lack of infrastructure, and the complexity of plastic types have resulted in lower recycling rates.
A well-established recycling infrastructure and policies could help achieve adequate plastic recycling and mitigate pollution.
From a sustainability standpoint, plastics are primarily derived from non-renewable sources like petroleum through polymerisation, which renders them unsustainable. The prevalent properties that make plastics desirable also contribute to their lack of biodegradability*.
Bioplastics: A promising replacement for plastics?
Natural elements such as heat, UV radiation, moisture, and oxygen can degrade plastic. They typically transform into microplastics (particles smaller than 5 mm) and nano plastics (particles smaller than 0.1 μm), posing significant risks to health and the environment.
Therefore, can plastics be derived from renewable* sources? Absolutely! Plastics can be derived from renewable, biological sources, known as 'bioplastics'.
Did you know that in the 19th century, scientists experimented with natural polymers like cellulose and rubber as animal-derived material' (ivory, horns, tortoiseshell) substitutes? In 1862, the first bioplastic was generated from cellulose!
Since then, several biological materials have been investigated for bioplastic formulation, persisting as an alternative even after the advent of synthetic plastics.
Great! So, bioplastics are a sustainable alternative as they are developed from renewable sources (such as plants and algae) and are biodegradable, are they not? No, not entirely! Bioplastics are either only 'bio-based' or 'bio-based and biodegradable'!
What Are Bio-based Bioplastics?
'Bio-based' bioplastics are made of polymers* derived from renewable biological sources (such as sugarcane or corn starch), entirely or partially. Bio-based polymers could be modified further to match the traditional synthetic plastics, structurally and property-wise.
However, like their synthetic counterparts, structurally modified bio-based plastics do not readily biodegrade in the natural environment.
Despite this, they offer advantages over fossil fuel-derived plastics by reducing reliance on non-renewable resources and lowering carbon emissions.
Coca-Cola Ventures into Plant-based Bioplastics
In 2015, Coca-Cola unveiled its prototype for a 100 % plant-based bottle. Coca-Cola has explored bottle options from B-PET (Bio-Polyethylene Terephthalate) to bPx (Bio-based Polyesters) crafted from bio-based polymers derived from sugarcane molasses and corn sugar.
It is worth noting that while these bottles are derived from plant-based materials, they are not biodegradable.
Are There Any Biodegradable Bioplastics?
Indeed, the bio-based plastics manufactured using chitosan, starch, lignin or cellulose polymers are biodegradable. Since the 1990s, starch-based bioplastics from crops such as corn, wheat, or potatoes and Polylactic acids (PLA) from corn starch or sugar cane have gained popularity due to their biodegradability and versatility.
An Animated Infographic That Depicts the Production of Bioplastic.
Microorganisms Produce Polymers for Biodegradable Plastics
Did you know that bioplastic polymers can be obtained from microorganisms? These microscopic organisms never cease to amaze us! Hence, the research on microbial-based, biodegradable-bioplastic polymers gained momentum as early as the 1970s.
Microorganisms have proven their ability to produce bioplastic polymers, polyhydroxyalkanoates (PHA)/ polyhydroxybutyrate (PHB). But when and why do these microscopic wonders synthesise and store these bioplastic polymers?
Exploring Microbial Bioplastics: Beyond PHA
Bacterial species are known for producing not only PHAs but also PLA. In addition to polyhydroxybutyrate (PHB), bacteria are capable of producing other bioplastic polymers such as poly(3-hydroxybutyrate) [P(3HB)] and polyhydroxybutyrate-co-valerate (PHBV).
Why Microorganisms Produce These Polymers
Microorganisms such as bacteria require the right proportion of nutrients for their growth, i.e., to increase in size and number.
When the carbon provided is surplus, and oxygen, nitrogen or phosphates are scarce, the microbes are stressed due to the unmet nutrient requirement.
The natural response of any organism in a stressful condition is to adapt and protect itself. Similarly, the stressed bacteria combat the situation by converting the excess carbon source to PHA polymers through metabolic pathways.
The synthesised PHAs are stored within the bacterial cells as their carbon and energy reserve.
An Animated Infographic That Describes the Conditions Required by the Bacteria to Synthesise the Bioplastic Polymers.
Microbial Bioplastics: How They Are Made
PHAs produced by the stressed microbes are categorised by their length. The most commonly studied form of PHA polymer, PHB (short-chain category), has properties similar to traditional synthetic polymer, polypropylene.
However, these polymeric reserves are present within the cell. How are they removed to be used as polymers for bioplastics?
Primarily, the growth of the selected PHA-producing microorganism is facilitated in large vessels called 'fermentors'. Fermentors are a 'growing haven' for microorganisms.
In these stainless-steel vessels, specific conditions such as temperature, pH level, oxygen supply, and nutrient availability are regulated to support optimal microbial growth.
Furthermore, for the microbial synthesis of PHA, scientists meticulously study and maintain conditions that encourage PHA production. This includes fine-tuning factors like temperature, pH balance, and nutrient levels to stimulate the desired metabolic pathways within the microorganisms, leading to efficient PHA synthesis.
For a sustainable and cost-effective approach, agricultural waste and by-products, starch and starchy wastewater, and effluents from oil and paper mills are utilised as the carbon-rich feed for fermentation.
These diverse carbon sources and additional nutrients fuel the growth of microbial cells capable of producing PHA polymers. After fermentation, these microbial cells are harvested.*
Once harvested, PHA polymers produced within the microbial cells are extracted by breaking open the cells. No need to fret! These bacterial cells have a short lifespan and are to perish within a few hours.
The extracted PHA polymers mix in the soup of cell debris and other impurities. Using a suitable solvent* that dissolves PHA, the polymer is separated from insoluble cellular debris.
Finally, the PHA polymers are recovered from the solvent and refined into a powder, pellet, or film that can then be converted into a bioplastic product.
An Animated Infographic Illustrating Microbial PHA Production and Its Downstream Process.
Making Bioplastic Production Cost-effective and Scalable
Presently, scientists contemplate employing genetically engineered microorganisms and inexpensive carbons to make biopolymer production cost-effective and scalable.
Genetic alterations result in bigger microbial cells that can produce and store bioplastic polymers in maximum quantities.
Also, efforts are ongoing to develop improved bacterial strains to refine extracellular* PHA production technologies for industrial applications.
Microbial Bioplastic Manufacturing: Current Status and Applications
Even after decades, the research on microbial bioplastics is escalating to enhance bioplastic polymer production. Today, a few biotechnology companies are pioneering technologies that promote sustainable and eco-friendly PHA production.
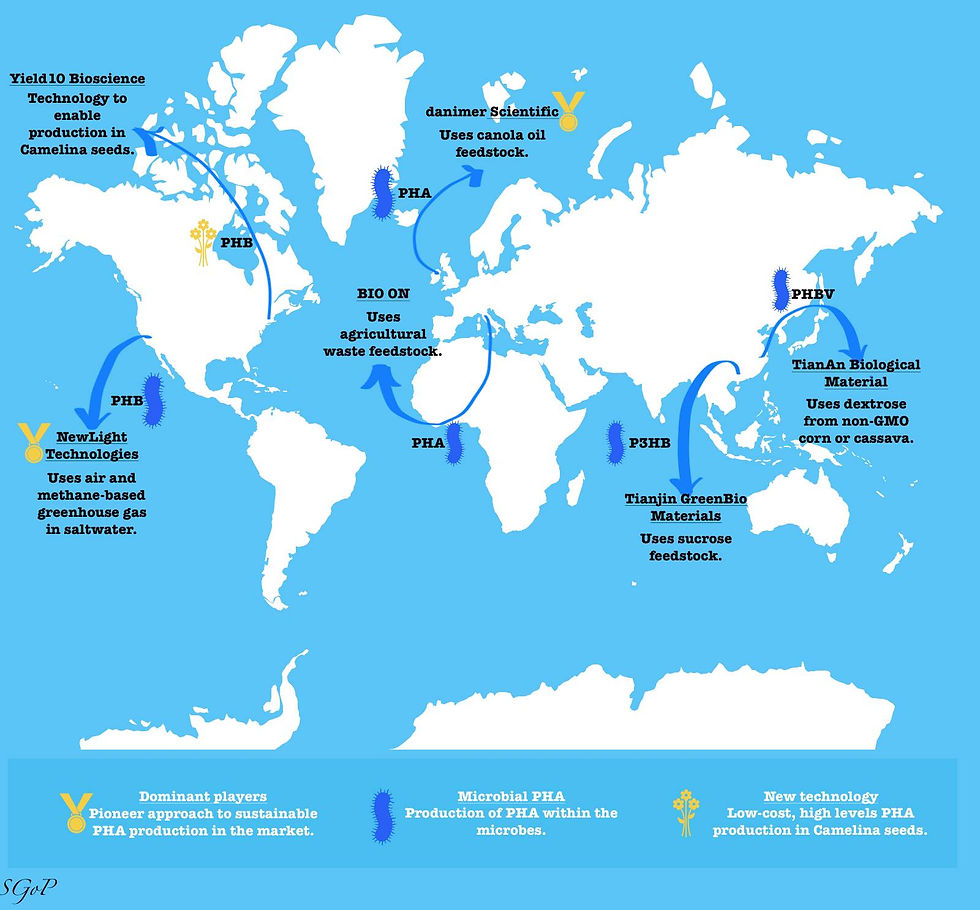
PHA is gaining traction as an attractive alternative to synthetic polymers. However, I was curious to explore if the transition of the PHA polymers to bioplastic products has been fruitful in the markets.
I was delighted to see the application of PHA in packaging and food services, biomedical, agriculture (weed-suppressing mulch films), fibre materials, and paper coatings.
However, the range of products developed by one particular company is outstanding. Bio-on has devised products independently or through converters* using Minerv-PHA, the brand name of the PHA manufactured by the company.
For the benefit of the readers, this company has made several PHA-based products: food packaging materials, cosmetics (sunscreen and micro-beads), sustainable toys, furniture, pharmaceutical capsules, and fashion fabrics and yarns that are 100 % bio-based and biodegradable.
Plastics from Renewable and Non-renewable Sources That Are Biodegradable or Non-biodegradable.
Bio-based | Non-bio-based | |
Biodegradable | Starch Polylactic Acid (PLA) Polyhydroxyalkanoate (PHA) | Polybutylene adipate terephthalate (PBAT) Polybutylene succinate (PBS) Polycaprolactone (PCL) |
Non-biodegradable | Bio-Polyethylene Terephthalate (Bio-PET) Bio-Polyethylene (Bio-PE) Polyethylene furanoate (PEF) | Polyethylene (PE) Polypropylene (PP) Polystyrene (PS) Polyethylene Terephthalate (PET) Nylon Acrylonitrile butadiene styrene (ABS) |
Final Thoughts
Are you pondering why conventional synthetic plastics are still being manufactured and used when promising alternatives are available?
The answer is multifold: First, the infrastructure for producing synthetic plastics and its supply chain is well-developed.
Secondly, the production costs involved with conventional synthetic plastics are cheaper due to the production scale and cheaper raw materials.
Third, there is a widespread preference for affordable and convenient synthetic plastic products over sustainable alternatives.
In retrospect, bioplastic production is catching up. Although starch-based bioplastics account for most biodegradable bioplastic manufacturing, global microbial bioplastic production is rising.
Continuous research on improved microbial strains and manufacturing technology has resulted in scalable, cost-effective PHA production.
Although it may not be possible to replace all the products that employ conventional plastics, it is the need of the hour to replace single-use synthetic plastics, especially food packaging.
Though recyclable, most often, owing to the greasy nature of these plastics contaminated with food residue or oils, they are unfit for recycling.
Most often, these plastics are subjected to incineration or landfilling. With biodegradable PHA bioplastics, home or industrial composting can degrade them into water, carbon dioxide and compost with the aid of tiny helpers, the microbes.
Do you think microbial bioplastics are safe alternatives to the conventional plastics? Share your thoughts in the comment box below.
*Concepts made simple
Biodegradable = The ability of a substance to be naturally broken down to its simpler forms, in addition to carbon dioxide and water. Microorganisms (bacteria, fungi) and other living organisms are the major contributors to the process.
Converter = A company specialising in modifying or combining raw materials to create products.
Extracellular = Outside the cell.
Harvest = The separation of the cells by centrifugation, filtration, or sedimentation.
Polymers = A substance comprising of repeating subunits (monomers).
Renewable = Naturally replenished or continuously available within a short duration.
Solvent extraction = Chemicals such as chloroform, methylene chloride or propylene chloride that can dissolve PHA polymers are used.
References
Bioplastics News | Bioplastics and Chemical Recycling news monitoring. (n.d.). Bioplastics News. Retrieved March 12, 2024, from https://bioplasticsnews.com/
Coca-Cola unveils new prototype bottle made from 100% Plant-Based sources | Press release. (2021, October 21). Retrieved March 7, 2024, from https://www.coca-colacompany.com/media-center/coca-cola-unveils-new-prototype-bottle-made-from-100-percent-plant-based-sources
Costa, A. F., Encarnação, T., Tavares, R. A., Bom, T. T., & Mateus, A. (2023). Bioplastics: Innovation for green transition. Polymers, 15(3), 517. https://doi.org/10.3390/polym15030517
Polyhydroxyalkanoate (PHA) market. (2023, October). Global Market Insights (GMI). Retrieved March 13, 2024, from https://www.gminsights.com/industry-analysis/polyhydroxyalkanoate-market
Samadhiya, K., Sangtani, R., Nogueira, R., & Bala, K. (2021). Insightful Advancement and Opportunities for Microbial Bioplastic Production. Frontiers in Microbiology, 12. https://doi.org/10.3389/fmicb.2021.674864
The Age of Plastic: From Parkesine to Pollution | Science Museum. (2019, October 11). Science Museum. Retrieved March 5, 2024, from https://www.sciencemuseum.org.uk/objects-and-stories/chemistry/age-plastic-parkesine-pollution
Varghese, S., Dhanraj, N. D., Rebello, S., Sindhu, R., Binod, P., Pandey, A., Jisha, M. S., & Awasthi, M. K. (2022). Leads and hurdles to sustainable microbial bioplastic production. Chemosphere, 305, 135390. https://doi.org/10.1016/j.chemosphere.2022.135390
Comentários